What Are Dark Matter And Dark Energy?
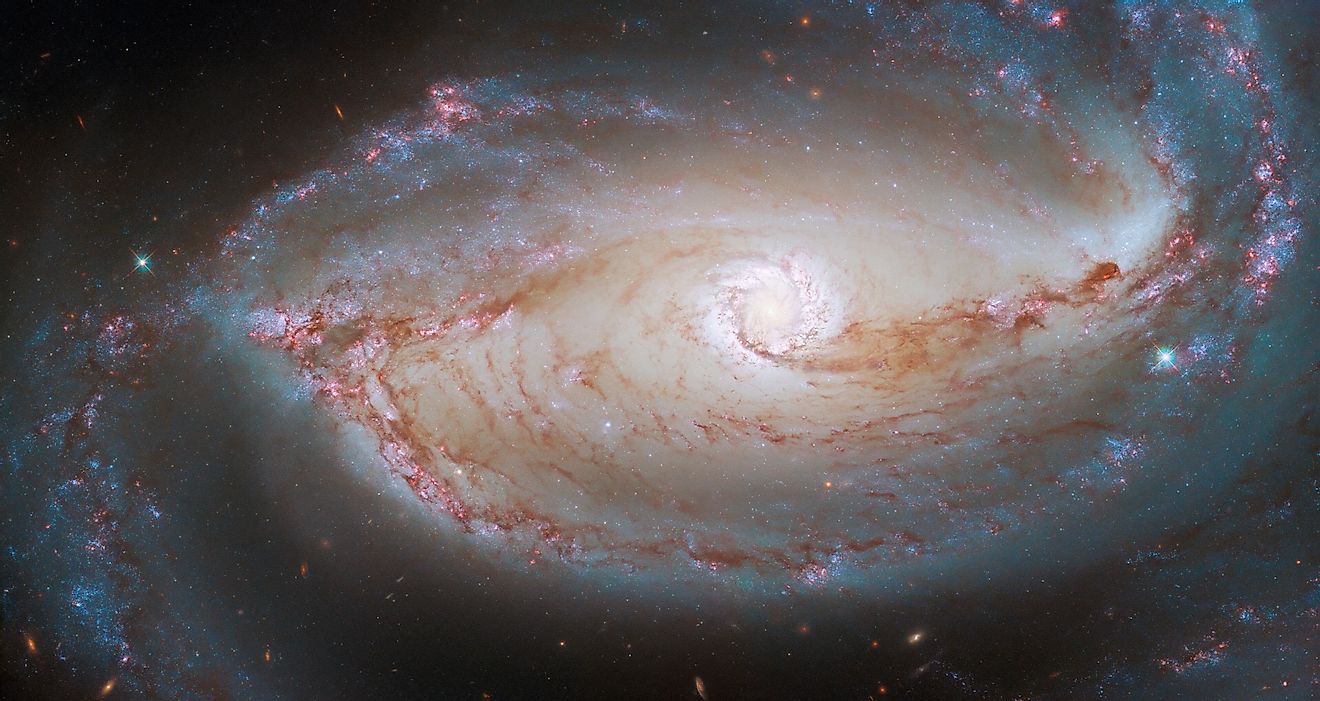
- Combined, dark matter and dark energy make up 95% of the total universe
- Dark matter is detected by its gravitational influence on visible objects
- Dark energy is causing the accelerated expansion of the universe
When we look out into space, we can see a universe full of stars, planets, and galaxies. Our eyes can see a lot, yet what we can see actually only comprises a fraction of what the universe is made of. Even with technology designed to overcome our limited senses, 95% of the universe is still invisible to us. That’s because most of the cosmos isn’t what we can see, but rather, is composed of two mysterious substances called dark matter and dark energy. Dark matter comprises roughly 27% of the total stuff in the universe, with dark energy making up 68%. That means that all the visible matter we can see, all the galaxies, stars, and planets, makes up a mere 5% of the total stuff in the universe. The rest of it is not only invisible, but we know virtually nothing about it. What do we know about dark matter and dark energy?
Dark Matter
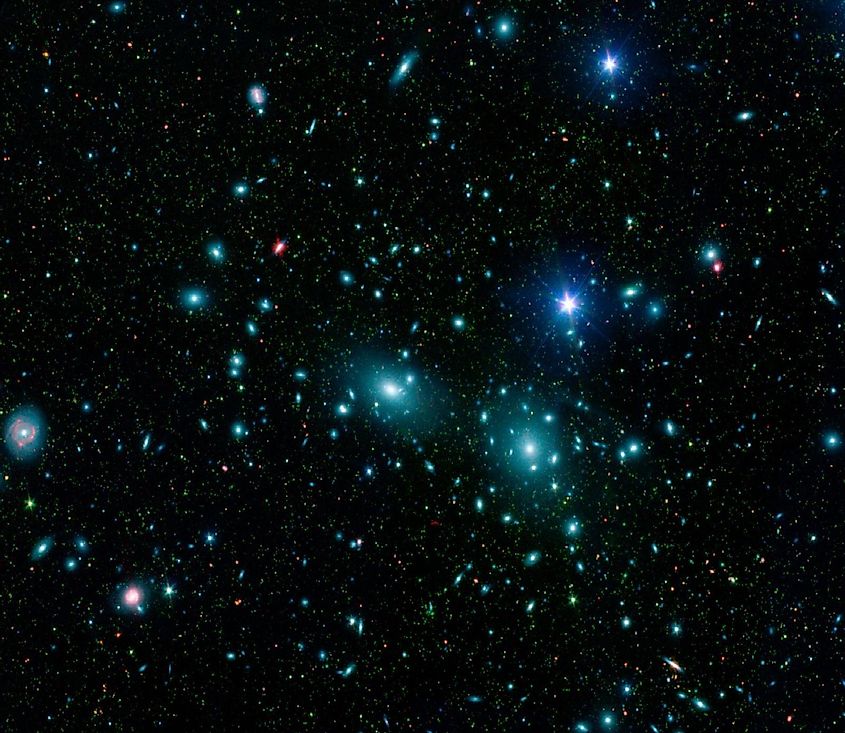
As previously mentioned, 27% of the stuff in the universe is composed of dark matter. The only aspect about dark matter that scientists truly know is that it exerts a gravitational force and it does not interact with light or regular matter other than through gravity. The existence of dark matter was first proposed by Swiss astronomer Fritz Zwicky in the year 1933. While observing a cluster of galaxies called the Coma Cluster, he sought to measure the cluster’s mass. Using the mathematics of gravity provided by Newton and Einstein, he measured the overall mass of the cluster. However, he quickly encountered a problem. The total mass from all the visible galaxies could not explain how the cluster holds itself together. According to his calculations, there simply was not enough mass to keep the galaxies together in a group. Based on the laws of physics themselves, most galaxy clusters should be falling apart, yet this is not the case. Zwicky went on to propose another explanation. He theorized the existence of some form of matter exerting a gravitational force that kept the galaxies together. This unknown form of matter became known as dark matter, and as the years went on, more evidence of its existence began to prop up across the cosmos.
In the 1960s, American astronomer Vera Rubin would make one of the most tantalizing and important discoveries in relation to dark matter. At a time when women were largely discouraged from pursuing careers in science, Rubin would become the first woman to operate the Palomer Observatory, where she would make one of the most important discoveries in cosmology. She set out to measure the rotational velocities of galaxies, which astronomers predicted would behave in a similar way to the planets in our solar system. The closer you get to the sun, the faster a planet completes an orbit. For example, Mercury completes one orbit every 88 days, while Neptune, the furthest planet from the sun, completes one orbit every 165 years. Like our solar system, it was predicted that stars closer to the centre of a galaxy would orbit faster than those in the outer regions of the galaxy. However, as is the case with much of science, nature defies our expectations. Rubin found that, for the most part, all stars seemed to orbit the galactic centre at relatively the same velocity. Given the distribution of mass in most galaxies, this should not be happening. Rubin realized that she had uncovered evidence of dark matter, with the gravitational pull it exerts causing stars to move faster.
Since dark matter’s discovery, scientists have puzzled over what it is. Perhaps, some thought, dark matter is simply the accumulation of smaller objects that we can’t see with a telescope, such as rogue planets, black holes, white dwarfs, and neutron stars. However, even when these objects are accounted for, the numbers simply don’t add up to how much dark matter is needed to hold the universe together. In more recent years, one line of evidence has proven to be the most convincing: gravitational lensing. This phenomenon was first described by Albert Einstein in his general theory of relativity. Einstein had proposed that the force of gravity itself is simply the warping and bending of space by objects with mass.
For example, the sun warps the space around it while the planets travel along the very curvature of space itself. Einstein realized that if this is truly the case, then even light will bend around objects as it travels along curved space. It was during a solar eclipse that Einstein’s theory of gravity was proven, as astronomers observed the light from background stars bend around the sun. This phenomenon is known as gravitational lensing, because like a magnifying glass, space itself can refract incoming light and both warp and enlarge distant objects. The magnitude of this warping can be used to determine the mass of the object that is bending the path of light. Hence, if 27% of the universe is dark matter, gravitational lensing should be a fairly common occurrence. With the launch of the Hubble Space Telescope, astronomers have uncovered gravitational lensing occurring across the universe. Like the discoveries of Zwicky and Rubin, gravitational lensing in some galaxy clusters could not be explained by the mass of visible objects. Rather, calculations revealed a hidden form of matter exerting a gravitational force. As of yet, astronomers know very little about dark matter. So far, every experiment devised to uncover dark matter has turned up empty handed.
Dark Energy
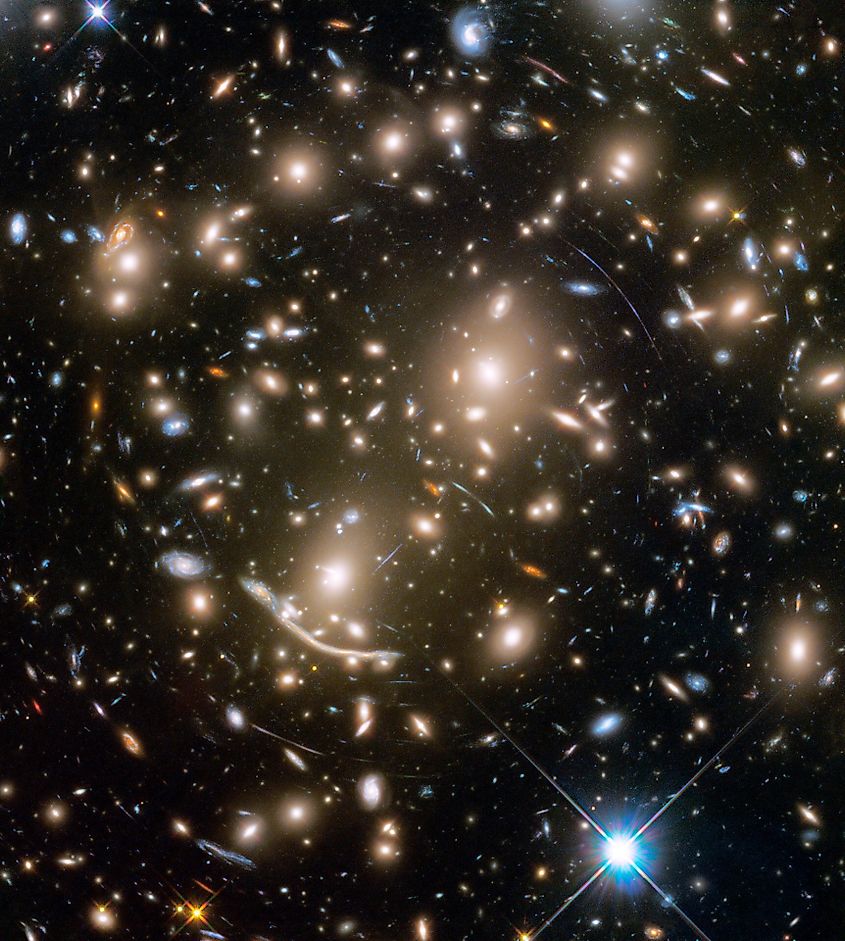
For as long as the universe has existed, space has been expanding. The cosmos is unbelievably vast, and space is only getting bigger. The universe today is larger than it was yesterday. If you rewind the clock far enough, you end up at a point where the entirety of space itself was compacted into a single point. The initial expansion of the universe was tremendous, yet it began to slow down in the couple billion years after the Big Bang. Understandably, scientists predicted that the current expansion of space would be slowing down. After all, it had become known that dark matter composed a very large portion of the universe, and so it was assumed that gravity would gradually slow down the expansion of space and the universe itself may start retracting in the distant future. To prove this assumption, two teams of astronomers set out to measure the rate of the universe’s expansion. In 1999, they both independently published their results and observed the same phenomenon. Rather than slowing down, space was speeding up. In order to determine how fast space was expanding, the two teams set out to find a way to determine distances to some of the furthest points in the universe.
Determining distances to the outer reaches of the observable universe is no easy task. In order to do so, they needed to find an object whose absolute brightness could be determined. In other words, they needed to find something that always gives off the same amount of energy. There aren’t many things that do this consistently in the universe, yet astronomers managed to find a process that always emits the same amount of energy. When sun-like stars run out of usable hydrogen, they evolve into red giants and eventually into white dwarfs. Interestingly, every white dwarf has an upper limit of how massive they can become. This limit, called the Chandrasekhar limit, is around 1.4 solar masses. Any white dwarf that happens to exceed this mass will undergo a runway thermonuclear reaction, resulting in a supernova explosion called a Type 1A Supernova. Because every white dwarf has the same upper mass limit, the amount of energy they give off will be identical regardless of the white dwarf. Although these types of supernovae are rare, the universe is large enough that astronomers have been able to find a lot of them.
The Doppler Effect
By knowing the exact energy output of the supernova, astronomers are able to determine the distance to the supernova. Furthermore, the light emitted by the supernova will be red shifted if it happens to be moving away from us. The Doppler Effect describes this observation by stating that waves of light can be stretched or compressed depending on what direction the object emitting that light is moving relative to us. When an object is moving towards us, its light becomes compressed and blue shifted. When it’s moving away from us, its light becomes stretched and red shifted. By measuring the red shift and distance to type 1A supernovae, astronomers are able to determine just how fast a galaxy is moving away from us. By doing this for multiple galaxies and supernovae, astronomers are able to determine the rate of the universe’s expansion. The data revealed an unexpected result: the rate of expansion is accelerating. Rather than slowing down as was predicted, the size of the universe is accelerating. However, in order for space to be accelerating, it must be permeated by some form of energy driving that acceleration. Kinda like how your car needs energy in order for it to start moving and more energy is required to get it accelerating. Astronomers named this energy dark energy, yet that’s about as much as they know about it.